2 Measuring and Extending DTV Coverage
Cable carriage of broadcast stations’ DTV signals is not mandated under current FCC rules as long as their analog station is carried. It appears, even after a station shuts down its analog transmitter, cable systems will not have to carry all the program streams the station chooses to broadcast. As a result, broadcasters are looking for ways to provide DTV service into areas where signal levels are inadequate for indoor reception or where over-the-air reception is blocked by terrain.
Two papers at the NAB2001 Engineering Conference outlined the promise and problems with on-channel DTV boosters in a single-frequency network configuration.
COVERAGE PREDICTION
For many years, the method most broadcast engineers used to measure coverage was the FCC F (50,50) graphs in Section 73.699, Figures 9 and 10, of the FCC rules. These propagation curves are based on a combination of actual signal strength measurements and calculations of field strength. The FCC’s allocation tables for analog TV were based on these curves.
When it became necessary to fit DTV channels into the same spectrum shared with analog TV, the FCC decided a more precise method of calculating coverage was needed and decided to use the Irregular Terrain Model (ITM) developed by the Institute for Telecommunication Sciences at the U.S. Department of Commerce. ITM is more commonly called the Longley-Rice model, after the scientists who developed it.
The FCC curves provide the distance to a field strength contour (or field strength at a certain distance) for a given power level at a particular height above average terrain (HAAT). HAAT is calculated by averaging the height of the center of radiation of the antenna over terrain 3.2 to 16.1 km from the antenna along the radial studied.
Longley-Rice, on the other hand, considers all terrain between the transmitting antenna and a specific receive point, so a coverage map will look like a checkerboard of cells (2 square km or less under FCC OET Bulletin 69) instead of a set of concentric contours. Because it more accurately accounts for terrain, it allowed the FCC to re-use channels in areas where terrain blocked interference.
PROPAGATION MODELS
To overcome some perceived problems with the Longley-Rice model, some consultants use another model, called "TIREM," that chooses among different propagation models based on the characteristics of the path to a particular point, avoiding the Longley-Rice "Error Code 3" problem.
William Meintel, in his paper "Television Service Predictions – Actual Measurements Versus Computer Modeling," compared the FCC curves, Longley-Rice (as implemented in FCC OET Bulletin 69) and TIREM with 2,937 measurements from stations on Channels 2 through 47 taken between 1998 and 2000 using the standard techniques described by Gary Sgrignoli for DTV field measurements. Data was taken from the recent MSTV-sponsored 8-VSB/COFDM study and from studies conducted in individual markets. These studies included paths over flat land, rolling hills and rugged terrain. Data was evaluated for "believability" before a measurement was used.
(click thumbnail)Figure 1
For the computer modeling, William Meintel used 3-second terrain data and 0.1 km terrain intervals. The antenna pattern, including the elevation pattern where available, was used in the calculations.
Overall, Longley-Rice predicted signal levels 7 to 17 dB stronger than the levels measured in the field tests. TIREM was slightly better, predicting signal levels 5 to 10 dB stronger than measured. TIREM did better on paths with fewer obstructions, whereas Longley-Rice performed better with more obstructions.
William Meintel concluded that the models were okay for "apples to apples" comparisons, but that they tend to over-predict actual signal strength. Longley-Rice and TIREM did better over longer paths.
INDIVIDUAL POINTS
Neither Longley-Rice nor TIREM should be considered valid when looking at individual points. Predictions for individual points showed a large standard deviation when compared with the field measurements, even for unobstructed paths. During the question-and-answer period, it was suggested that variations in land use (local buildings, foliage, etc.) could have accounted for the large errors on unobstructed paths.
(click thumbnail)Figure 2Clearly, models that are more complex are needed if we want more accurate coverage prediction. Some propagation software for land-mobile and cellular requires land-use data. In fact, point-to-point coverage studies for determining coverage under the U.S. Satellite Home Viewers Improvement Act require land-use data. This information is readily available. RadioSoft’s ComStudy software allows adding land-use attenuation to its Longley-Rice studies.
Figures 1 and 2 compare the field strengths predicted for WRC-DT at 813 kW ERP by Longley-Rice both with and without land-use attenuation. As you can see, land use does affect coverage. Both figures were calculated using OET-69 parameters for Longley-Rice coverage, with land use attenuation added in Figure 2. Cell size was 15 seconds, approximately 500 meters square. The OET-69 default elevation pattern was used.
ON-CHANNEL DTV BOOSTERS
Two papers at the NAB2001 Broadcast Engineering Conference examined on-channel DTV boosters. Multiple transmitters on the same frequency providing coverage of an area are referred to as a "Single Frequency Network" (SFN). Sam Zborowski, from ADC Telecommunications, covered SFN basics and the use of synchronization to reduce interference between on-channel transmitters operating on the same frequency in his paper "Single Frequency Network Technique For Use With On-Channel Boosters for DTV Broadcast." The next day, S. Merrill Weiss examined 8-VSB single frequency networks in more detail and outlined changes in the FCC rules needed to allow broadcasters to implement them in his paper "FCC Technical Rule Changes to Support Digital 8-VSB Single Frequency Networks."
When a DTV receiver is presented with two signals carrying exactly the same datastream on the same frequency, if the time difference between the dominant signal and the weaker one is within the correction capability of the receiver’s adaptive equalizer, the weaker signal will be treated as multipath. However, if the time difference is too large, the weaker signal will be treated as noise.
If the second signal is treated as multipath, a receiver’s adaptive equalizer can decode the data even if the difference between the two signals drops to only a few dB. Otherwise, if the second signal is treated as noise, reception will be impossible if the difference is less than 15 dB.
Sam Zborowski outlined the problems created by "leading" ghosts. Leading ghosts occur when the direct path to the TV transmitter is obstructed and the stronger signal arrives by reflection. This is common in indoor reception environments, particularly in urban areas, where the window closest to the antenna may not be facing the transmitter site but another building. In the case of an on-channel booster, there are often areas where a slightly weaker signal from the main transmitter arrives before the signal from the on-channel booster.
SFN DESIGN
When designing a single frequency network, the goal is to keep the delay between the two signals within the equalizer range of DTV receivers in the desired coverage area. This can be done either by increasing the equalizer range in the receiver or by synchronizing the data from the transmitters so that all signals arrive at the DTV receivers within their correction range.
Zborowski noted that early DTV receivers were able to handle leading echoes within about 2 to 3 microseconds of the dominant signal, although newer designs extend this to 5.9 microseconds. I have heard some new designs have equalizers with a total correction range of about 50 microseconds that handle much longer leading echoes by allowing the correction window to slide either side of the main signal.
Multipath can also be reduced by synchronizing the DTV transmitters in the SFN. GPS can be used to lock the frequencies of the transmitters within 1 Hz. DTV receivers won’t have a problem with this as they are designed to handle frequency shifts of several hertz caused by Doppler shifts from reflections off moving vehicles and airplanes. Synchronizing the data, however, is more difficult because of a startup ambiguity in the trellis coding in the ATSC modulator.
If the same ATSC MPEG transport stream is applied to two modulators, they could end up transmitting two different data streams. Even if the two signals arrived at the DTV receiver with a delay spread within the range of its equalizer, one of the signals would be treated as interference.
DATA RANDOMIZATION
One solution to this is to apply the data randomization, Reed-Solomon encoding, interleaving, trellis encoding and multiplexing functions to the transport stream before sending it to multiple transmitters. Because of the overhead of the extra information added to the transport stream, a data rate of more than 30 Mbps is needed to distribute it.
Another option being considered is a modification of the ATSC standard to allow transmission of a synchronizing signal that would periodically initialize the trellis encoders to the same state. This would simplify distribution but would require modifications to existing DTV exciters.
Zborowski used a design being considered by WPSX for a DTV booster network in western Pennsylvania to demonstrate a large improvement in coverage when the required desired-to-undesired (D/U) ratio could be reduced from 20 dB to 2 dB. A D/U of 20 dB is appropriate where the delay between the two transmitters can’t be equalized in the receiver. A D/U of 2 dB is a realistic value for newer DTV receivers where the second signal can be treated as multipath and equalized. In the first case, coverage was very spotty, with about half the area of interest not able to receive a signal. In the second case, only a few areas had interference.
S. Merrill Weiss outlined the need for on-channel boosters and single frequency networks. He pointed to studies showing the higher signal levels, up to 80 dBu, needed for indoor reception. An SFN offers the benefit of shorter interference zones compared to a single high-power, high-elevation transmitter, particularly when coverage is needed in communities distant from the transmitter. Multiple transmitters allow diversity reception, filling in holes in coverage, improving performance with set-top antennas and helping mobile and pedestrian reception.
Before broadcasters can begin implementing single frequency networks to improve coverage, some FCC rules must be changed. Weiss filed comments with the FCC requesting that on-channel boosters be considered primary users under Part 73 of the FCC rules. A coverage extension up to 50 percent in each direction should also be allowed.
Boosters located within a station’s contours using the facilities specified in the FCC DTV Table of Allotments should be allowed as an alternative to maximization using high-power transmitters. Booster power limits, however, should be the same as those for the main station. Coverage from both main and booster facilities should be considered together when defining service area and they should be treated as a single source for interference purposes, to avoid double counting interference to other stations from overlapping SFN transmitters.
Weiss said he had requested the SFN rules be given expedited consideration and considered separately from the translator and LPTV DTV rule makings. In addition to the FCC changes, Merrill Weiss has proposed the ATSC standardize synchronizing information that can be transmitted in the ATSC signal to precisely control the delay from on-channel transmitters in the SFN.
If the availability of reliable DTV reception, especially at UHF, is going to approach that of VHF analog coverage, SFN techniques will be required in many areas. It will be interesting to see if the FCC allows broadcasters to take full advantage of these techniques and if broadcasters follow through and install multiple transmitters in an SFN.
Contact Doug Lung via e-mail atdlung@xmtr.com
Get the TV Tech Newsletter
The professional video industry's #1 source for news, trends and product and tech information. Sign up below.
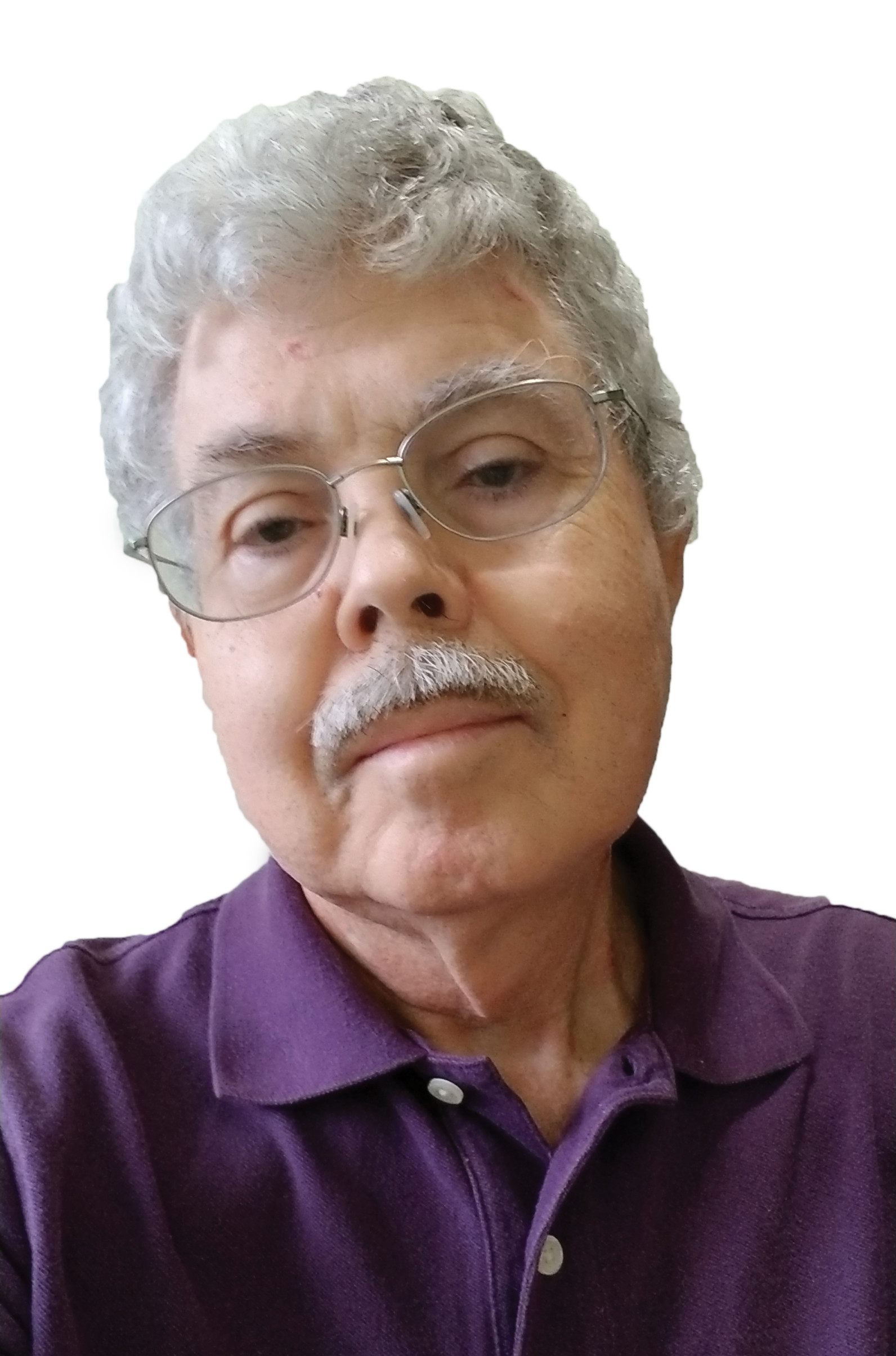
Doug Lung is one of America's foremost authorities on broadcast RF technology. As vice president of Broadcast Technology for NBCUniversal Local, H. Douglas Lung leads NBC and Telemundo-owned stations’ RF and transmission affairs, including microwave, radars, satellite uplinks, and FCC technical filings. Beginning his career in 1976 at KSCI in Los Angeles, Lung has nearly 50 years of experience in broadcast television engineering. Beginning in 1985, he led the engineering department for what was to become the Telemundo network and station group, assisting in the design, construction and installation of the company’s broadcast and cable facilities. Other projects include work on the launch of Hawaii’s first UHF TV station, the rollout and testing of the ATSC mobile-handheld standard, and software development related to the incentive auction TV spectrum repack. A longtime columnist for TV Technology, Doug is also a regular contributor to IEEE Broadcast Technology. He is the recipient of the 2023 NAB Television Engineering Award. He also received a Tech Leadership Award from TV Tech publisher Future plc in 2021 and is a member of the IEEE Broadcast Technology Society and the Society of Broadcast Engineers.