Combining digital and analog TV signals
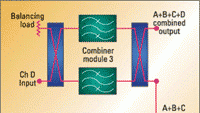
Broadcasters seeking to implement digital television (DTV) know that it is critically important to introduce this service in the timeliest and most cost-efficient manner without reducing the performance of existing analog services.
One way to do this is to add an additional transmitting antenna onto a tower already carrying an analog antenna system. But, unless a rigorous site analysis indicates no other option for a specific site, this method may present problems. Not only does a second antenna system impose a considerable additional weight load onto an existing tower (leading to possible structural modifications), but also it can also result in uneven coverage. It can even introduce propagation conditions whereby the protection ratio between the two signals is altered differentially, creating interference between the DTV and analog services.
Figure 1. This diagram shows three balanced combiners cascaded to form a multichannel combiner chain. Each combiner consists of a balanced pair of filters, two 3dB couplers and a balancing load.
A better alternative is to use RF combining technology, which permits multiple services to operate from a common antenna. Forming an operational duo with broadband panel antenna arrays, RF combiners can play an integral role in achieving combined analog and digital service. This approach makes the best possible use of existing site hardware while ensuring uniform coverage and minimizing interference.
Adjacent-channel challenges
But combining UHF analog and digital television services presents its own challenges. Many of these stem from the problem of combining so-called “adjacent channels.” Spectrum authorities around the world prefer this technique for analog/DTV in their quest to optimize spectrum use. In conventional analog broadcast applications, interference problems previously rendered the “gaps” between semi-adjacent channels unusable. The advent of digital broadcast has liberated these gaps, allowing broadcasters to use adjacent channels. But, to combine contiguous channels, RF engineers have had to develop sophisticated, higher-order filters.
Broadcasters seeking to overlay existing analog services with digital television are likely to encounter a variety of combining scenarios. One broadcaster might have to add a single digital service to a single analog channel, while another might have to combine 10 or more contiguous or semi-contiguous channels in varying combinations of digital/analog or digital/digital.
Whatever the specific situation, each broadcaster must consider a range of practical issues. Many of these are specific to those systems where channels are adjacent. These issues include overcoming the challenge of narrow guard bands, optimizing insertion-loss characteristics and correcting for group delay. Other issues, such as filter performance and emission masking requirements, are common to both adjacent and semi-adjacent channel combining.
Basic principles
There are four main classes of combiners: starpoint, manifold, commutating line and balanced. Of these, the balanced combiner has the widest range of applications due to its modular construction and minimal interaction between inputs. Its purpose is to combine multiple transmitter signals into a single antenna system while keeping the transmitters isolated and properly matched.
A balanced combiner module consists of a balanced pair of filters, two 3dB couplers and a balancing load. The transmitter signal enters the narrowband input, while the channels previously combined — or another single-channel — enters the wideband input. The combined output is directed either to the antenna itself — or to the wideband input of the next module in a cascaded multichannel combiner. (See Figure 1.)
Overcoming narrow guard band
The fundamental challenge of using adjacent channels is squeezing many signals into a limited amount of spectrum. To compensate for a very small band gap between channels, combining adjacent channels centers around the concept of partially band-limiting signals and then applying pre-correction to the transmitters to compensate.
The challenge is particularly severe for cases where the lower channel is analog. The size of the guard band depends upon system types, but is typically on the order of a couple of hundred kHz. For example, consider a coded orthogonal frequency-division multiplex (COFDM) signal (channel N) and a PAL signal located just below it (channel N - 1). In this case, the separation between the PAL signal’s B stereo sound carrier and the lower portion of the COFDM spectrum is just 170kHz. To overcome the potential system degradation resulting from this narrow guard band, a practical solution widely implemented with COFDM systems is to raise the frequency of the digital channel by 100 to 150kHz. Experiments have shown that the resulting encroachment into the vestigial sideband of an upper analog channel (N + 1) has little effect, provided other signal corrections are applied.
Optimizing insertion loss
Insertion loss is the amplitude response of an RF signal through a module. Ideally, the signal is constant or zero; in practice, it is distorted non-uniformly across a channel. For adjacent channels, the distortion is especially severe because of the tight channel spacing and small guard bands. Combining adjacent RF channels, therefore, requires special “sharp” tuning of the individual combiner modules to ensure the whole cascaded system operates at optimum performance.
Within any given adjacent-channel combiner module, if the channel entering the narrowband port is tuned to experience a 3dB attenuation at the upper and lower frequency edges, attenuation at the wideband port (normally inverse) will also equal 3dB. This is the optimum situation for adjacent-channel combining, assuming an ideal, lossless filter.
Figure 2. Tuning a filter to achieve optimum response.
Figure 2A shows response of channel N through such an ideal filter. Narrowing the filter in this way is sometimes referred to as “sharp tuning.” But, if an adjacent channel signal (N-1) passes into the wideband port of the combiner module meant for channel N, the upper edge of channel N-1 will be attenuated by a further 3dB, resulting in a total attenuation of 6dB. (See Figure 2B.) You can avoid this situation by tuning the combiner module to make the upper 3dB point of each narrowband port higher in frequency than the upper edge of its applicable channel. Figure 2C shows the widened response of the channel N-1 signal within its own module. Passing channel N into the combiner module then achieves optimum response. (See Figure 2D.)
Normally, the passband limiting described here would severely attenuate the upper adjacent channel. You can avoid this by introducing the channels in descending order of frequency, starting with the highest frequency channel at the output.
Overcoming group-delay distortions
Associated with the amplitude distortion introduced by the sharp-tuned filters is a nonlinear phase response in the signal —known as group delay —which, in turn, requires correction in most systems. Group delay manifests itself in both DTV systems (8VSB and COFDM), but in different ways. With the COFDM system, the filter itself attenuates some of the DTV extreme carriers and requires a slight increase in transmitter power to compensate. On the other hand, correction is vital for the 8VSB standard, as the signal is considerably more sensitive to group-delay distortion. All 8VSB adjacent-channel systems require adaptive equalization within the transmitter, involving the combiner in a continuous feedback loop.
The most common way to correct group delay for the two analog systems (PAL and NTSC) is to introduce a pre-distorted signal into the transmitter. This method is known as IF correction. Particularly stringent correction of the NTSC signal is required when the upper adjacent channel is digital (usually 8VSB) because the aural carrier of the analog channel is extremely close to the new digital channel.
Optimizing filter performance
At the heart of any balanced combiner is a pair of filters whose principal role is to combine the signals from multiple transmitters while cleaning up any spurious transmitter emissions. To meet digital/analog channel combining requirements, higher-order filters for both waveguide and coaxial combiner systems are available.
Cross coupling also has proven an important design element for conventional wide and semi-adjacent combiners. This technique works by introducing one or more additional signal paths to connect non-adjacent resonators, thereby producing frequencies of zero transmission as well as normal bandpass characteristics. Cross-coupled filters provide the best characteristics for a given number of resonators.
Because increasing the filter selectivity by increasing its order also increases the loss at the band edges, the challenge is then to select the filter that provides the optimum combination of selectivity and loss. (Figure 2 shows that the best theoretical filter response for adjacent channel combining is a -3dB response at the channel edge.) The other performance criterion to consider is the amount of group delay, which becomes more severe with the sharper roll-off characteristics and flatter, wider pass bands that distinguish higher-order filters.
Figure 3. This diagram compares the selectivity, loss and group-delay characteristics of a 6-pole cross-coupled filter with that of an 8-pole filter.
Figure 3 compares the performance of 6-pole, cross-coupled coaxial filters with standard 8-pole coaxial filters. While Figure 3A shows that the two filters have very similar selectivity characteristics in the critical region at the channel edges, Figures 3B and 3C show that the 6-pole, cross-coupled coaxial filter has lower loss and group-delay characteristics. In other words, the 6-pole, cross-coupled coaxial filter is sufficient to meet the adjacent combining requirements of most systems, obviating the need to migrate to larger, more expensive 8-pole filters.
Emission masking
In addition to combining adjacent-channel signals from multiple transmitters, high-order filters can also mask spurious transmitter emissions. Transmission authorities generally legislate standards defining the maximum out-of-band energy radiated by the transmitter and filters. In other words, the sum of the transmitter response plus the filter response must fall within this mask.
Although broadcasters can accommodate masking requirements within the transmitter, they are becomingly increasingly aware that the combiner system itself fundamentally provides the additional filtering required to comply with these requirements. This obviates the need for building additional filtering equipment into the transmitter.
To achieve the sharp masking response required for adjacent- and semi-adjacent channels in combined DTV/analog applications, you can carefully tune cross-coupling paths to produce “notches” or cross-coupling zeros in the filter characteristic. While you can tune conventional analog filters using simple iterative techniques, the DTV filter demands a far more systematic, prescribed approach, such as that provided by computer simulations. This approach allows you to consider a greater number of variables, leading to a much more sophisticated design.
Graham Broad is the broadcast area product manager for Radio Frequency Systems.
Get the TV Tech Newsletter
The professional video industry's #1 source for news, trends and product and tech information. Sign up below.